Research in Advanced Materials
Problem Statement
Progress in science and industry needs the knowledge, production and utilization of newly customized materials such as laminated composites, nanocomposites, porous and functionally graded materials. Smart material solutions are developed while incorporating self healing, active piezoelectric and shape memory characteristics. At ARL-MLS, we focus on developing the aforementioned solutions while providing resilient, eco-friendly and biocompatible solutions to industries. Advanced material exploration and investigative methodologies such as atomistic, micromechanics, multiscale modelling are enacted towards characterization and improvement of material properties for applications in aerospace, biomedical and engineering structures. Our goals are to overcome the following challenges such as:
- Structurally efficient polymers and elastomers for lightweight structures with functionality.
- Industry specific material identification and selection.
- Sustainable eco-friendly materials for advanced structures and devices
- Discovering new materials for bioengineering applications.
- Identification and selection of replaceable fillers for high performing composites and nanocomposites.
- Characterizing the lifecycle of newly developed materials.
- Mapping effective processes for easy manufacturability and utilization of advanced materials.
Solutions
To overcome the many global challenges in the field of Materials Engineering, ARL – MLS has been actively developing cutting edge solutions such as:
- Physical characterization of new materials tailored towards industrial applications.
- Discovering Multi dimensional properties of materials for additive manufacturing (4D Printing / 5D Printing).
- Developing tailored multifunctional materials for sophisticated engineering applications (porous, nanocomposites, piezoelectric and flexoelectric materials).
- Resilient and robust material solutions to withstand extreme environments.
- Cost effective self-healing material alternatives for extending structural life.
- Development of composite based fillers to accentuate performance of conventional materials.
- Developing customized functionally graded materials for lightweight multifunctional structures.
- Generating new material alternatives for shock absorbance and damping of projectile impacts.
Projects
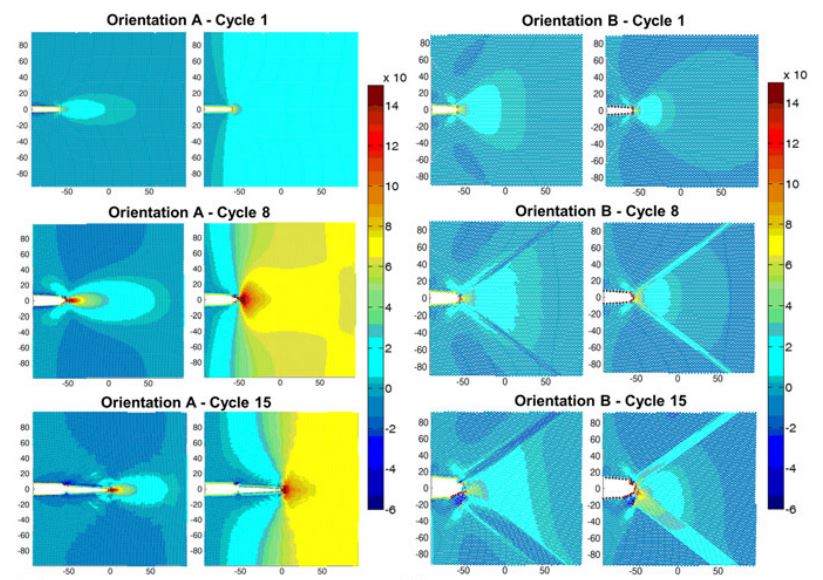
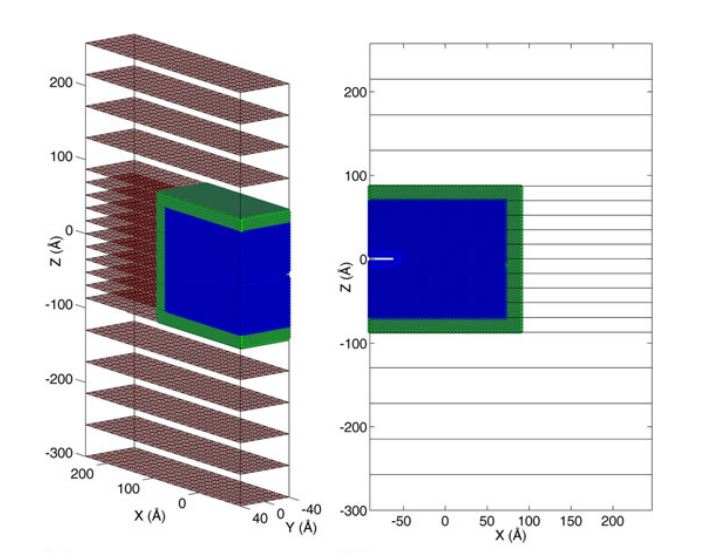
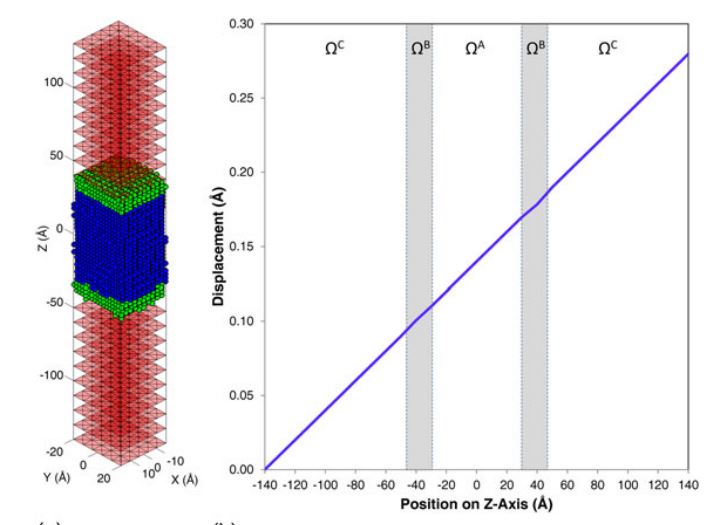
Abstract
The previously developed bridging cell method for modeling coupled continuum/atomistic systems at finite temperature is used to model fatigue crack growth in single crystal nickel under two crystal orientations at different temperatures. The method is expanded to implement a temperature-dependent embedded atom method potential for finite temperature simulations avoiding time-scale restrictions associated with small timesteps. Results for the fatigue simulation were compared with respect to deformation behavior, stress distribution, and crack length. Results showed very different crack growth mechanisms between the two crystal orientations as well as reduced resistance to crack growth with increased temperature
Citation
Iacobellis, V., & Behdinan, K. (2015). Bridging cell multiscale modeling of fatigue crack growth in fcc crystals. International Journal for Numerical Methods in Engineering, 104(13), 1200-1216.